Project Area C:
Engineering hybrid quantum systems
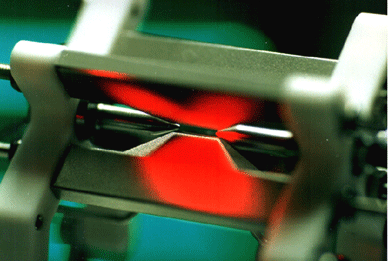
Quantum hybrid devices are engineered by combining methods and ideas from the fields of solid state physics, quantum optics, and atom optics. Here, recent advances in nanofabrication lead to completely new types of quantum devices. Often, these devices require engineering of long phase memory times and well controlled interactions between the building blocks. Project area C aims to use a combination of systems and ideas from quantum/atom optics and solid state physics to foster the development of new quantum devices. This effort will combine the activities of the two communities not only conceptually but also to develop real devices within the framework of the SFB.
The common subjects in this section, light-matter and atom-solid coupling are used to generate, control and probe specific quantum states of matter. Engineered solid-state systems are used for the design of quantum states and simple atomic quantum systems are employed to investigate solid state properties.

Projects C2 and C5 investigate micro-structured traps for atoms. While C2 deals with large samples of neutral atoms, which are loaded into a magnetic trap and eventually undergo Bose-Einstein condensation, project C5 investigates micro-Paul traps which confine single atomic ions. The common impetus of both experiments is the investigation of a quantum optical system - atomic BEC and a string of single ions - with a nearby cryogenic solid state surface. Both projects contribute to the goal of engineering hybrid quantum systems where atoms are coupled to a solid state system in order to probe the influence and the effect of decoherence.

The central goal of project C2 is the application of a micro fabricated magnetic trapping potential generated by superconducting stripes at cyrogenic temperatures for the coherent manipulation of atomic gases and Bose-Einstein condensates. These lithographically fabricated wave guide potentials may be regarded as a single mode fiber for atomic waves. There are several reasons why we develop superconducting micro traps. First, niobium superconducting stripes will allow us to overcome the well studied disturbing effects on the atomic BEC caused by conventional conductors. Of particular interest in project C2 are the spin coherence time and its behaviour when crossing the normal to superconducting transition, Casimir-Polder forces between atoms and the surface, and the interaction of atoms with magnetic vortices. Another aim is the coupling of atoms to superconducting devices, such as Josephson-junctions and SQUIDs. While in C2 the coupling between atoms and solids is due to the atomic magnetic moment, in C5 the atomic system - a single ion or a string of trapped ions - interacts via Coulomb forces with electric charges on the nearby trap electrodes.
In project C5, ultra-cold trapped ions or ion crystals in engineered quantum states are realized for a systematic study of interactions with condensed matter systems. The ions are used as a probe of the decoherence caused by a nearby surface. Detailed studies of the interactions include a variation of the surface temperature, the ion-surface distance, the surface preparation, and the investigation of superconducting materials such as niobium, or high Tc-materials for a superconducting segmented micro ion trap. The ions will be lowered to the surfaces either by using micro-structured three-dimensional or planar segmented traps. The influence of the surface as a source of decoherence will be investigated using ground state cooled ions in a superposition of internal or vibrational quantum states, and entangled ion crystals. In planar devices, we intend to integrate micro- and nano-objects, eventually nano-mechanical oscillators, which are sensed by the ions nearby. In the long run, we plan to shrink the size of the trapping structures and objects which are coupled to the ions, in order to engineer - as envisioned similarly in project C2 - macroscopic quantum states via a mutual interaction with the ions. This will lead to the development of quantum sensors and fundamental studies of decoherence of macroscopic quantum states. In addition, project C5 establishes a close relationship to the projects in area B, as linear strings of ions are well suited to simulate quantum phase transitions, benefiting from the high degree of control and the efficient read-out of multi-ion entangled quantum states by means of laser-ion interaction. Apart from the close collaboration with project C2, also a strong link exists to C9 with neutral atoms trapped close to nanostructures. The quantum optics aspect of the project leads to a collaboration with C4 on the generation of entanglement using laser light fields.
The understanding of the energy level structure, exciton formation and coherent control of quantum states in quantum dot molecules is in the focus of project C3. The optical properties and the coherent control of single quantum dots has been subject of intense research. Quantum dot molecules however are significantly more difficult to produce and hence little is known about the coherent evolution of their excited states. By precise control over the deposition process it is possible to preadjust the coupling mechanism between two individual quantum dots. By embedding the molecular quantum dots in electrically gateable devices, the electronic structure of the quantum dot molecule is completely externally adjustable. The goal of project C3 is to study the fundamental electronic and coherent optical properties of vertically and laterally coupled quantum dot systems.

The long term goal is to achieve a precise control over the state evolution in quantum dot molecules and over the scaling in multi-quantum dot structures in order to achieve control over large quantum systems. The project uses a number of optical techniques to study the coherence times in ground state quantum dots as well as in their exited state. The project has close links with project C4, where defect centers are brought into interaction with each other. The differences come from the much larger electron correlations in defect centers which make them a more atom-like system with point-like wavefunctions where electron and holes always reside at the same defect center. The projects C3 and C4 share a number of complementary goals which will stimulate transfer of knowledge between two different communities in solid state physics. In principle, the quantum dot system also has two different kinds of interaction, the exchange and the dipolar interaction, which might be tunable with respect to each other similar to what is intended in C4 and C5, and was explored in the ongoing project A3.

Project C4 aims to expand quantum control techniques pioneered in quantum optics to create tunable and coherently controllable mesoscopic spin ensembles. The creation of such "large scale" ensembles is a central challenge in the field of synthetic quantum systems. We will use NV vacancy defect centers in diamond to study the scaling from few to many-body properties. In these systems, improvements in material quality and novel optical addressing techniques present a realistic way towards the generation of coherently interacting spin arrays. One possible approach for the coupling is to utilize the strong interaction with the photon field and a subsequent beam splitter to entangle distant defects. Alternatively, the mutual dipolar interaction between spins can be used to generate arrays of coherently interacting defects. This latter approach provides a strong link to the projects studying dipolar interactions in polar molecules B8, interacting Rydberg atoms B6, and cold atomic gases A2. In project C4 we will also study the coupling of individual defect centers to a two-dimensional surface conduction layer of diamond. This work will establish a connection to the studies - of surface generated decoherence in C2 and C5. Possible applications of NV ensembles also provide a link to the quantum emulation of condensed matter systems: a spin coupled to a charged system may be used to investigate Kondo physics by placing spin impurities with high accuracy in the ensemble. The coherent control of the mesocopic quantum systems studied in this project is also a goal of projects C3 and A1, and offers important opportunities for collaboration.
The scientific objective of project C9 is to observe the interaction of ultracold atoms and carbon nanotubes. The main focus is on the realization of nanomechanical resonators based on carbon nanotubes, the cooling of nanomechanical resonators by scattering cold atom beams on nanotubes, and the construction of cold atom - nanomechanical hybrid quantum systems. Goals of the project are tailoring new dynamic cooperative quantum states and controling cold atom- nanodevice interactions. The dominant interaction between the atoms and the carbon nanotubes is the Casimir-Polder interaction, which will be experimentally derived from scattering data of ultracold rubidium atoms transported in an integrated magnetic conveyor belt from the carbon nanotubes. In a second part, the mechanical vibrations of the nanotube are examined and the potential for cooling them via a cold atom beam is studied. For this purpose, the nanomechanical resonators based on carbon nanotubes will be mounted on the surface of a cryostat, which allows one to remove a large number of oscillation quanta from the mechanical resonator via a precooling before scattering cold atoms from it. Evidence of further cooling by the cold atom beam would have significant consequences for pushing mechanical resonators towards the quantum regime. The cold atom - nanoresonator system is a promising candidate for constructing novel hybrid quantum systems. An intense collaboration is intended between A1 and C9 with respect to the fabrication of high quality nanostructures and to low-temperature measurement techniques provide a strong link to the experimental groups in C2 and C5. Theoretical overlap is expected with A7 with respect to the manipulation of atoms by microstructures, while interaction with respect to experimental techniques used with cold atoms is intended with A2, B6, C2 and B7.