Project Area B:
Controlling Quantum Phase Transitions

Quantum phase transitions are a fundamental concept of condensed matter physics. They are a ubiquitous mechanism that can lead to novel states; prominent examples are hightemperature superconductors and heavy-fermion materials where clear deviations from the canonical description of metals (Fermi liquid theory) are observed. The term quantum phase transitions refers, in general, to phase transitions that occur at zero temperature (T=0 K). Therefore they are controlled by a parameter other than the temperature (like doping, pressure, magnetic field), such that the ground-state is changed in some fundamental way. A second, very interesting aspect is that the critical behaviour is dominated by quantum fluctuations due to zero-point motion. As a consequence, the dynamical and the static properties are intrinsically coupled for a quantum phase transition. This is in contrast to classical phase transitions, where the critical behaviour is driven by thermal fluctuations. Since quantum phase transitions cannot be simply controlled by regulating the temperature as in classical ones, it is general rather difficult to monitor them experimentally in conventional condensed matter systems. Tailored quantum matter offers an opportunity to overcome such difficulty in atomic gases as well as in the solid state.
We expect that in project area B, a close interaction of experts from atomic physics, quantum optics, and condensed matter will lead to a fundamental understanding and control of quantum phase transitions both in equilibrium and in nonequilibrium. Starting with an understanding and control of interparticle interactions provided by the expertise in atomic physics, we expect to reveal the scaling behaviour going from few-body to manybody systems relying on methods and systems generally encountered in condensed matter physics. In closely related realizations with atoms, molecules and solids, where both quantum optics and condensed matter expertise play a fundamental role we expect to find new states of matter and to tailor dynamically cooperative quantum states.

Project B4 aims at the study of non-equilibrium properties of strongly correlated systems. The project will deal with systems that originally reside in the areas of quantum gases and condensed matter. Due to the non-perturbative nature of strongly correlated systems, numerical techniques like quantum Monte Carlo simulations or Density Matrix Renormalization Group (DMRG) are the natural tools to deal with. In the first period, a time dependent Density Matrix Renormalization Group (t-DMRG) was implemented. This numerical tool will be applied in the second period to time dependent Hamiltonians. One of the main focuses is on cold atomic fermions in time dependent optical lattices. Such a system will be experimentally realized in project B7, and a strong cooperation between the two groups is naturally given.

In Project B6, strongly interacting Rydberg gases are studied within an experimental and theoretical collaboration. The Rydberg states are coherently excited from an ultra-cold atomic gas. In such a setup, Rydberg gases are an ideal system to study the scaling from few body to many body physics: while the Rydberg state itself is a single particle property, the strong Rydberg-Rydberg interactions gives rise to blockade phenomena for small clusters of atoms. Increasing the atomic density then pushes the gas into a strongly interacting many-body system. During the time evolution of the driven system complex many-body states are formed. Present experiments show that the system saturates into a steady light-matter state. This steady state can be viewed in terms of a phase diagram featuring a second order quantum phase transition exhibiting universal scaling behavior with critical exponents, which are experimentally accessible. This observation provides a strong link of the project to the conventional description of quantum phase transitions in condensed matter physics. However, the strongly interacting Rydberg system presents a novel state of matter and its properties will be examined theoretically and measured experimentally. In addition, the equilibration of the system during time-evolution is a key point in the description of the system and provides a strong link to project B4, where nonequilibrium phenomena are extensively studied. The internal structure of Rydberg atoms is closely related to the rotational structure of polar molecules studied in project B8. Further, the interaction in both cases is also driven by a dominant dipole-dipole interaction. Since many properties and phases are studied theoretically in polar molecules this concept can also be applied to Rydberg atoms.
New states of matter are also explored experimentally in Project B7. The aim is to study two-component mixtures of cold atomic gases in an optical lattice. The system combines the successful tools of Feshbach resonances between the two atomic species and an optical lattice to obtain a highly controllable and tunable setup. In the first part, onedimensional standing waves are applied to the degenerate mixture of Rubidium and Lithium atoms near an interspecies Feshbach resonance, and its properties are studied with Bragg-spectroscopy. In the second part, the quantum gas is substantially modified by time dependent optical lattices. In a special type of two-dimensional lattice with rotating potential hills even more interesting physics is expected, including a novel type of quantum phase transition. The application of a time-dependent optical lattice imprints a timedependent phase onto the trapped quantum gas, which starts to develop a phasemodulated order parameter with d-wave symmetry. Of special interest is the realization of this setup with fermions, which then models the situation of d-wave order parameters in solid state physics far more realistically. The project is performed in close collaboration with the project B4, and is therefore another example of a fruitful collaboration between atomic physics and quantum optics experiments with condensed matter theory. The expertise gained by the theoretical study of the dynamics of the strongly correlated system in B4, will directly help in interpreting the experimental results, and will eventually culminate in the experimental realization of novel quantum states of matter. In addition, the cold atomic mixtures of rubidium and lithium with an interspecies Feshbach resonance in an optical lattice are promising candidates for the realization of ultra-cold polar molecules, which are studied theoretically in project B8.
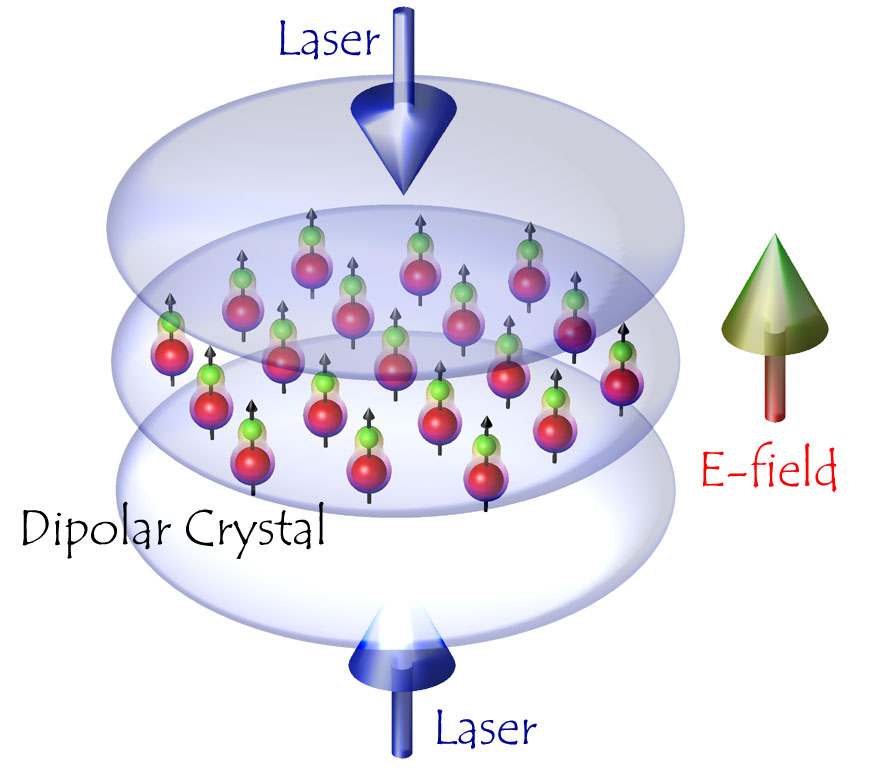
The central goal of project B8 is to study ground state properties and quantum phase transitions of ultra-cold polar molecules driven by external fields in the strongly interacting regime. Polar molecules have attracted a lot of interest due to their strong electric dipole moments, which allow for the realization of strongly interacting many-body systems. The focus in this project is then to explore theoretically the potential of polar molecules for creating novel quantum states of matter. The expertise from atomic physics and quantum optics, which allows us to control and tune single polar molecules and their interactions, is combined with the theoretical expertise in condensed matter physics for the understanding of the ground state properties of the system and their numerical analysis with quantum Monte Carlo simulations. The project shares the common goal with project B4 to develop state of the art numerical tools. In addition, due to the close relation between the internal structure of a Rydberg atom and polar molecules, a strong collaboration with project B6 is envisioned. For example the experimental expertise in coherently/adiabatically driving the system can be essential for the preparation and realization of the novel states of matter with polar molecules.
Beyond the strong and close link within area B, there are several links with projects in areas A and C. On the technical side, project B4 is connected to project A6, where similar numerical tools are developed and applied, while the non-equilibrium aspect of the system is an important part of project C4 for the manipulation of quantum states at defect centers. Dipole-dipole interaction present between Rydberg states discussed in B6 and polar molecules in B8 are also experimentally studied in project A2 for magnetic interaction between chromium atoms.
In project B6 also a direct connection exists to the project A7, where interactions between ionic impurities in a quantum gas are studied. The theoretical treatment of those impurities in A7 will possibly be applicable in this project. In addition, there is a significant overlap with the goals of project C3 where collective excitonic excitations in quantum dots and quantum dot molecules will be investigated. The concept of mesoscopic spin systems, where a mesoscopic number of spins form a collective quantum system is directly related to project A3.
The project B7 is closely connected to project A2, where bosonic and fermionic mixtures are also discussed in the context of dipolar gases. Intense exchange of experience concerning the technology around ultra-cold gases takes place with projects C2 and C9 which is located at the same institute in Tübingen. The project B8 is connected to A2, which is also aimed at the experimental creation of "quantum emulators" in the context of cold atomic gases. The aspect of coherent manipulation of spin systems is common to the projects B8 studying polar molecules, B6 on coherently driven Rydberg atoms and project C4 on quantum states in defect centers.